top of page
How to analyze thermal radiation in nonlinear photonics systems? Why is it challenging?
How to analyze thermal radiation from generic bianisotropic media
Radiative heat transport phenomena have been extensively studied using common isotropic materials. Very recently, other classes (which are computationally difficult to analyze) such as uni/biaxial anisotropic materials and gyroelectric materials are explored. Our goal is to develop tools and techniques to extend this exploration to many other unexplored classes of nontrivial materials like gyromagnetic, magnetoelectric, and other nontrivial materials that have not been explored yet.
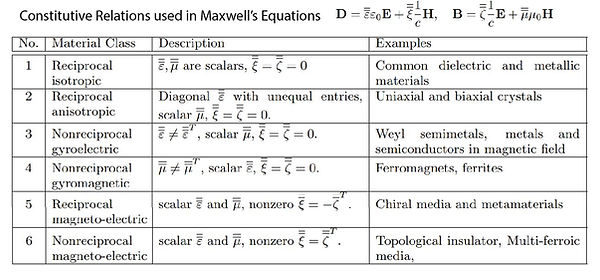
In our recent works, we have found interesting 'photon-spin'-related phenomena using gyrotropic and magnetoelectric materials. See fundamenal prospects page.
A universal look at thermal-radiation properties has also enabled us to discover new spin-resolved Kirchhoff's laws. We believe that this research direction will be promising in the long run.
New J. Phys. 2019
New J. Phys. 2020
Gain-loss systems for controlling and enhancing near-field radiative heat flux
Thermal radiation and near-field heat transfer are described using fluctuational electrodynamic theory. We have generalized it to describe incoherent amplified spontaneous emission from gain media below lasing threshold.
We show that a combination of loss-compensation (using gain medium) and near-field interactions can be utilized to obtain and tailor giant (orders of magnitude enhanced) radiative heat flux at the nanoscale. Because of a large magnitude of heat flux (comparable to conductive flux rates), this finding will be important for nanoscale heat management.
Phys. Rev. B. 2016

Spintronic approach for controllable nonreciprocity and spin-photonic applications


We propose a design (left schematic) which uses strong spin-orbit-coupling (SOC) of a narrow band-gap semiconductor InSb with ferromagnetic dopants. A combination of intrinsic SOC with gate-applied electric field leads to strong external Rashba spin-orbit-coupling. This makes the magnetically doped InSb thin film a gyromagnetic medium (non-symmetric permeability tensor).
We then show photon-spin-dependent Purcell effect in the vicinity of this thin film which has gate-controllable nonreciprocity. As shown in the figure, a dipole (classical source or atomic/molecular transition) emitting right circularly polarized light experiences larger Purcell factor than that emitting left circularly polarized light.
Phys. Rev. B. 2020
bottom of page